ABSTRACT:
Excimer laser angioplasty
was developed in the early 1980s in an ef fort to solve two of the limitations
of balloon angioplasty, recanalization and restenosis. Excimer lasers ("cool"
lasers) generate nanosecond, high power, UV pulses that strip electrons
from the nucleus of an atom. This causes bonds to break and plaques to
vaporize with shock waves or photoabolative effect. In its best essence,
laser angioplasty technology offers the potential for moving a fiberoptic
catheter through the entire length of the coronary circulation to vaporize
all plaques along the arterial wall. While thermal damage has been sufficiently
reduced in excimer laser angioplasty, this procedure is still used only
in minority of the cases as a stand-alone operation due to high-pressure
waves and bubble formation caused by excimer laser.
INTRODUCTION:
Serious stake in laser angioplasty
began in the early 1980s in an effort to solve two of the limitations of
balloon and stent angioplasty, restenosis and recanalization. By demonstrating
the capability of laser irradiation to vaporize atherosclerotic tissue,
it was reasonable to presume that this new technology may allow recanalization
of lesions that could not be crossed by conventional balloon insertion.
With refinements in laser fiber optics, FDA approved excimer laser procedure
in 1992 for clinical use as recanalization device in both coronary and
peripheral arteries. However, the requirement to follow laser angioplasty
with balloon angioplasty in the majority of cases and the insufficiency
of an effect of laser tissue removal on restenosis has limited the usage
of this procedure. Perhaps, improved techniques discussed later in this
paper such as better fiber-optic lens systems or saline fusion will allow
laser angioplasty to offer a true niche in interventional cardiology (Ref.
6). This paper has been written to give a general overview of laser angioplasty,
its procedures, and its applications.
TEXT:
Angioplasty is a method used
to open coronary arteries blocked by obstructing plaques. Plaque by definition
is a build up of cholesterol and other fatty molecules inside an artery.
Over time, plaques can slow or even stop the flow of blood to and from
the heart. In order for the heart to function properly, the normal flow
of blood must be retained, by cleaning or bypassing the blockage. One procedure
used to remove a plaque is laser angioplasty. In laser angioplasty, a thin
tube (a catheter) is inserted into an artery and moved through the blood
vessels to the blocked artery. The laser emits short pulses of photons
that cause the plaque to vaporize. Alternative methods for removing a plaque
from an artery include coronary artery bypass surgery, mechanical removal
surgery, and balloon angioplasty. The method used depends on the location
of the plaque, size of the plaque, and the number of plaques. Laser angioplasty,
however, is still not used alone very often (Ref. 1). Typically, it is
used with balloon angioplasty. When a plaque has totally blocked an artery,
laser angioplasty can be used to drill a hole in the plaque, so the balloon
angioplasty can be successfully performed. Therefore, laser angioplasty
has become a safe and a cheap alternative for expensive, bypass, open-heart
surgery (Ref. 1)
Laser angioplasty does not
replace balloon angioplasty, however, when used with the balloon catheter,
more patients can be operated successfully at low medical costs. Today,
the usage of laser and balloon angioplasty has become so popular that bypass
operation is barely used. So what exactly is balloon angioplasty? In balloon
angioplasty, the cardiologist inserts a long, hollow, narrow tube, catheter,
into an artery through an incision made in the groin or the arm. Using
the X-ray technology, the doctor can monitor the exact place of the catheter
inside an artery. The cardiologist advances the tube through the blood
vessels until he gets to the blocked area of the artery. The second thinner
catheter is then inserted into the first hollowed tube. At the tip of the
second catheter exists a small miniature, a deflated balloon. Once in proper
position, the miniature is inflated, causing the narrowed area of the artery
to become slightly wider. In some cases, a device known as stent is inserted
via another narrow catheter. Stent reduces the likelihood that the artery
becomes narrowed again (Ref. 1). Figure 1 illustrates the usage of balloon
and stent.
Figure 1: Usage of Balloon
and Stent (Ref. 10)
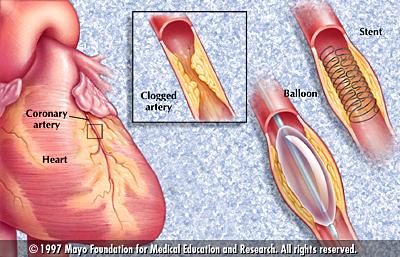
As Figure 1 shows, balloon
angioplasty can be used only when the miniature is placed inside the plaque.
If the plaque has completely blocked the artery, then before inserting
the balloon catheter, a laser catheter must be inserted to drill a hole
through the plaque to open a space for the miniature.
In order for laser angioplasty
to be a successful operation, it is essential that a laser with the right
wavelength and energy be chosen. The laser should remove the plaques without
damaging the blood cells or the blood vessel tissues. Thermal damage caused
by the laser energy being absorbed by the tissues depends on the wavelength
of the laser, duration of the beam, tissue color, consistency, and water
content. As the tissue absorbs the laser energy, more and more heat energy
is produced. The temperature continues to rise over 100 C. This will cause
the protein denaturization in the cells and cause the water inside the
cells to evaporate. Since water vapors occupy more volume than the liquid
water, the cells burst and the cell membranes break open. To minimize this
thermal damage, short pulses of laser is instead used which reduces the
heat energy produced by the radiation; therefore, decreases tissue damage
(Ref. 1). Table 1 shows the biological and visual changes that takes place
as the temperature increases.
Table 1: Tissue Changes
with Temperature
Temperature (C) |
Visual Change |
Biological Change |
37-60 |
None |
Warming, welding |
60-65 |
Blanching |
Coagulation |
65-90 |
White/Grey |
Protein Denaturization |
90-100 |
Puckering |
Drying |
100 |
Smoke plume |
Vaporization, carbonization |
As Table 1 notes, the laser
used in angioplasty should produce a temperature of about 65-90 degrees.
At this temperature, protein is denaturized, atomic and molecular bonds
are broken, and plaques can be removed. At higher temperatures, thermal
damage of the tissues is expected while at lower temperatures, plaques
cannot be removed.
There are three different
methods of laser angioplasty: thermal, photothermal, and photoablative.
In thermal laser angioplasty, an argon, or Nd: YAG laser is used to heat
a biocompatible metal ally tip attached to a fiberoptic waveguide. The
highest temperature is 400C, which is the temperature of the very tip of
the probe. The surgeon guides the probe through the obstruction causing
a small lumen for the blood to flow through. This method is not very useful
since it can cause thermal damage of the tissues and carbonization and
necrosis of the vessel walls. Photothermal laser angioplasty involves a
contact of a Nd: YAG laser probe that rapidly heats the plaque to the point
of vaporization using a photo-optical effect. This method is more productive
and safer than the thermal method, but still can cause damage to vessel
walls. The third method is photoablative laser angioplasty that involves
the usage of excimer laser. The excimer, excited dimer, laser uses either
two noble gas atoms or a noble gas atom in conjunction with a halogen atom
to lase at different wavelengths, mostly Ultraviolet (Ref 3). Table 2 shows
different examples of excimer laser and their output wavelengths.
Table 2: Examples of Excimer
Lasers and Their Output Wavelength (Ref. 3)
Excimer
|
Wavelength (nm)
|
Excimer
|
Wavelength (nm)
|
ArF |
193 |
bbF2 |
157 |
KrF |
248 |
Kr2 |
146 |
XeF |
351 |
Xe2 |
72 |
XeCl |
308 |
KrCl |
222 |
ArO |
558 |
KrO |
556 |
XeO |
558 |
|
|
The energy source of an excimer
laser can be a beam of few electrons at high energy or many electrons at
high current discharge (100,000 A). XeCl is the most common excimer laser
that is used for angioplasty. In XeCl laser, an electron collides with
a Xe atom causing it to go to an unstable electron configuration, which
allows it to bind with a Cl atom and produce XeCl. Since there are no XeCl
molecules at a low energy state, population inversion takes place (Ref.
3).
Xe + Cl ======>
XeCl + Cl-
e- + Cl2 ======>
Cl- + Cl
Cl- + Xe+
+ M ======> XeCl + M
Gases used in excimer lasers
typically have short lifetimes; therefore, they must be refilled constantly.
Lifetime of gasses used in some of the excimer lasers have been outlined
in Table 3.
Table 3: Excimer Laser
Properties (Ref. 3)
Excimer Gass
|
Fill Lifetime
|
ArF |
10^6 shots |
KrCl |
10^7 shots |
KrF |
10^6 shots |
XeCl |
10^7 shots |
XeF |
10^6 shots |
Excimer lasers require special
UV mirrors and optics to operate. Mirrors and lenses of these lasers apply
fused silica, magnesium fluoride, or calcium fluoride substrates with aluminum
or magnesium fluoride coatings. The excimer laser generates nanosecond,
high power pulses that strip electrons from the nucleus of an atom. This
causes bonds to break with shock waves or photoabolative effect. The laser
light is fired in short blasts through fiber optic bundles. This laser
method, “cool” laser, used since 1988, became the first laser to be approved
by FDA for angioplasty in 1992. The precise accuracy of these pulses of
UV light is made possible by series of magnetic switches developed by the
Jet Propulsion Laboratory. This method is so precise that it can remove
exactly 9 millionths of an inch of tissue in 12 billionths of a second
(Ref. 1). One example of laser angioplasty is Smooth Excimer Laser Coronary
Angioplasty (SELCA) which uses a XeCl laser with the following properties:
Pulse Duration
= 115 ns
Repetition Rate =
100 Hz
Wavelength = 308 nm
Area of radiation
= 1 mm2
Energy Density = 50
mJ/mm2
Energy of Pulse =
50 mJ
The frequency of this XeCl laser
can be found using Equation 1.
f = c/lam
(Equation 1)
f = frequency of light
lam = wavelength of
light
c = speed of light
Speed Of Light = 3
* 10^8 m/s
f = (3 * 10^8 m/s)
/ (308 nm * 10^-9 m/nm) = 9.703 * 1014 s-1
The energy of a photon emmited
by this laser can be found using Equation 2.
Ep = hf (Equation
2)
Ep = engergy of photon
h = plank’s constant
f = frequency of light
Plank’s Constant =
6.626 * 10^-34 J.s
E = (6.626 * 10^-34
J.s) * (9.703 * 10^14 s-1) = 6.43 * 10^-19 J
The number of photons emmited
in each pulse can be found via Equation 3.
N = Et / Ep
(Equation 3)
N = number of photons
emmited in each pulse
Et = total energy
of pulse
Ep = energy of photon
N = (50 mJ * 10^-3
J/mJ) / (6.43 * 10^-19 J) = 8 * 10^16
The rate of laser energy delivery
is called power and is measured in watts. The power of XeCl laser can be
calculated by Equation 4.
P = E * R
(Equation 4)
P = power
E = energy of each
pulse
R = repetition rate
P = (50 mJ) * (150
Hz) = 7500 mW = 7.5 W
Power density, or irradiation,
is the amount of power that is concentrated into a spot. The power density
can be calculated using Equation 5.
D = P / A
D = power density
P = power
A = spot size
D = (7.5 W) / (1 mm2)
= 7.5 W/mm2
The excimer laser generates
less heat than thermal and photothermal lasers. This reduces the carbonization
and thermal damage of the blood vessels. While safer, excimer laser method
still raises some safety concerns. The gases that are used for this method
are typically very toxic and even fatal. No leakage of these gases can,
therefore, be allowed. The UV radiation generated by the excimer laser
can also cause genetic mutations of the DNA by breaking the hydrogen bonds
between the two strands. Overall, laser angioplasty is also associated
with many potential problems including, including reocclusion, vascular
spasm, perforation, cardiac arrhythmias, and intimal dissection (Ref. 3).
To increase the precision
of the operation, the physician must be able to distinguish the plaque,
blood cells, and vessel walls. Chromophore-tagged monoclonal antibodies
that selectively attach to plaque molecules have been developed. When the
plaque is tagged, only the tagged molecules absorb the therapeutic laser
beam. Therefore, the chance of damaging the surrounding vessel walls has
been greatly decreased (Ref. 1).
Studies have shown that during
XeCl excimer laser ablation of tissue, small, short lived bubbles, predominately
containing water vapors, are formed that can damage the blood vessel tissues
(Ref. 2). These rapidly expanding and imploding bubbles are less than 3
mm in diameter and have a lifetime shorter than 300 microseconds. For a
given radiant exposure, the size of these vapor bubbles can be decreased
either by decreasing the surface area of the tip of the fiber or by reducing
absorption coefficient of the hemoglobin solution (Ref. 5).
CONCLUSION:
From 1988 to 1998, approximately,
14,000 patients were treated with excimer laser angioplasty (Ref. 4). The
data underline the workability and safety of this procedure. However, laser
angioplasty so far has been used as a stand-alone technique only in minority
of the cases. Clinical studies have also shown that excimer laser angioplasty
does not reduce the incidence of restenosis. While safer, laser angioplasty
still can injure vessel walls by high-pressure waves or bubble formation.
Technical and procedural progress including homogeneous light distribution,
infusion of saline, and the concept of smooth laser ablation have been
pushed forward to make excimer laser angioplasty safer, more predictable,
more precise, and more effective.
Researchers continue to work
for the development of new laser methods to be used in angioplasty. Promising
new lasers are expected to have less thermal tissue damage. Solid-state,
pulsed-wave, mid-infrared (2.1 microns) holmium:YAG laser has recently
been used for angioplasty. It is still too early to predict the success
rate of this new laser technology (Ref. 8).
REFERENCES:
-
Ball, K. Lasers; The Perioperative
Challenge. Mosby - Year Book, Inc. St. Louis,
Missouri. 1995.
-
Dawson, P., Booth, A. and Machan,
L. Laser angioplasty: enough to make your blood
boil! Br J Radiol (ENGLAND),
1994 Apr, 67(796):346-8.
-
Gerald, D. and Darrell, L. Understanding
Lasers. Futura Publishing Company, Inc.,
Mount Kisco, NY. 1991.
-
Haase, K. and Karsch, K. Coronary
laser angioplasty: clinical value and experimental
progress. Z Kardiol (GERMANY),
1996, 85 Suppl 1:81-6.
-
Leeuwen, T., Velema, E., Pasterkamp,
G., Post, M. and Borst, C. Saline flush during
excimer laser angioplasty:
short and long term effects in the rabbit femoral artery.
Lasers Surg Med (UNITED
STATES), 1998, 23(3):128-40.
-
Leeuwen, T., Jansen, E., Welch,
A. and Borst, C. Excimer laser induced bubble:
dimensions, theory, and
implications for laser angioplasty. Lasers Surg Med
(UNITED STATES), 1996, 18(4):381-90.
-
Sanborn, T. Laser angioplasty:
historical perspective. Semin Interv Cardiol
(ENGLAND), 1996 Jun, 1(2):117-9.
-
Topaz, O. Holmium laser angioplasty.
Semin Interv Cardiol (ENGLAND), 1996 Jun,
1(2):149-61.
-
http://www.mech.gla.ac.uk/~davidb/lectures/laserapp/angiop.htm#I1
-
http://mayohealth.org/mayo/9307/htm/angiopla.htm
http://www.spectranetics.com/vitc2a.html
|